Canonical protein kinase structure and motifs
Overview
Gly-loop
VAIK/α-C helix
HRD motif
DFG motif
Miscellaneous
Overview of a typical protein kinase structure
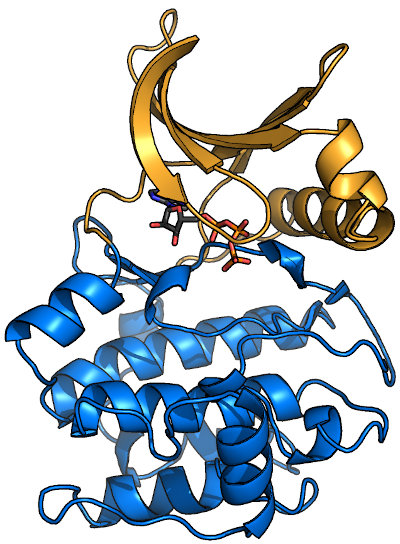
Protein kinases (like PKA, shown here bound to ATP1ATP) form a clamshell-like
fold that clamps down on substrate. The N-terminal half of the protein (or N-lobe; orange in image) is predominantly β-sheet, while the C-lobe (blue)
is mostly α-helical. Most kinase domains range in molecular weight from 30-40 kDa, and many kinase subfamilies have specific insertions. Most, but not
all protein kinases evolved from a single common ancestor. Those that did not are known as "atypical" kinases, e.g. α-
kinases, Rio, and lipid kinase relatives, such as TOR and its orthologs. Such atypical kinases are currently not represented in this database.
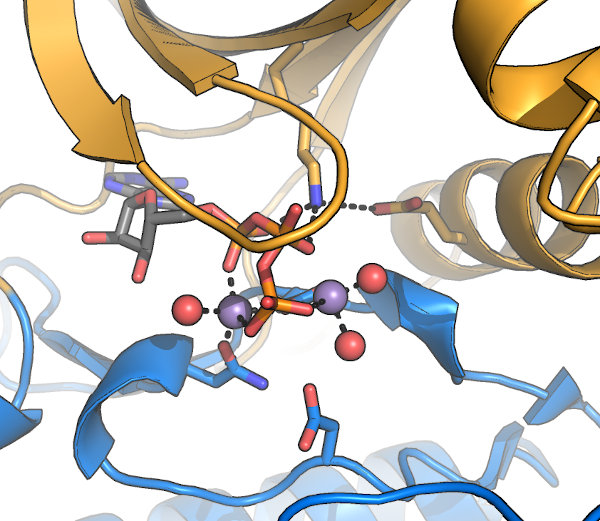
The kinase active site: A set of conserved motifs are required to coordinate 2 bound Mg2+ and the nucleotide required
for activity. These motifs were first described by Hanks & Hunter, and often referred to
by their "Hanks & Hunter subdomain" number. Note that some contacts are made indirectly, via water molecules (red in this image). Other conserved motifs mediate the
actual reaction, while additional residues, sometimes distal from the active site, are involved in kinase activation and substrate interaction.
Some kinase-fold proteins have naturally occurring substitutions in residues required for activity. Such proteins are known as
pseudokinases, and may be involved in regulating the activity of other enzymes (including true kinases). In a few cases, new catalytic activities
have been described for apparent pseudokinases.
The Glycine-rich loop (P-loop)
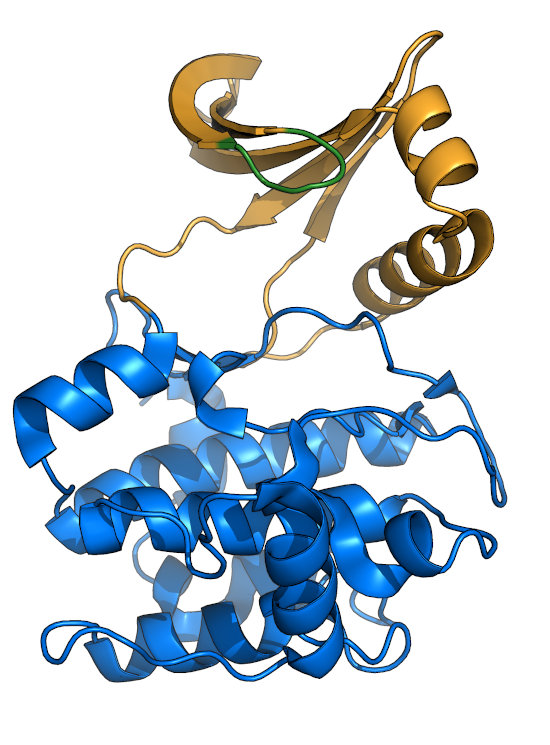
The Gly-rich loop (green), also called the P-loop or Walker A motif, consists of the motif "GxGxxG".
The center glycine is the only residue thought to be required for nucleotide-binding, as some kinases retain activity with substitutions
at other positions. The Gly-loop is quite dynamic, and clamps down upon binding of nucleotide (see comparison between
apo1J3H
and ATP-bound1ATP
PKA structures, and is often unresolved in crystal structures of apo kinases. While catalytically inactive pseudokinases often have
degraded Gly-loops, both the Apicomplexan FIKK and WNG families appear to have
lost the Gly-loop structure, but are still highly active kinases.
The VAIK motif and α-C helix
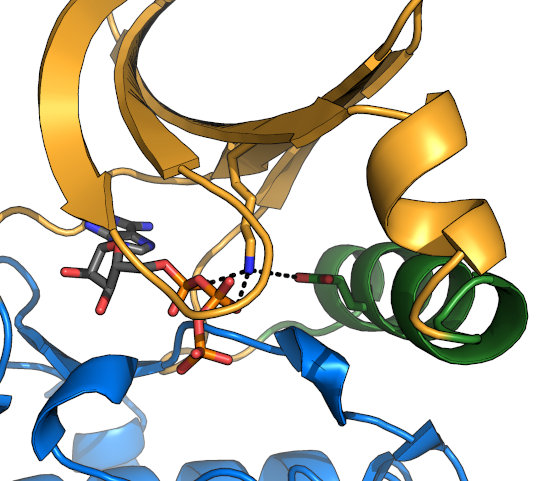
The "VAIK" motif sits in β-sheet 3 of the kinase N-lobe. The lysine (K)
in the motif interacts with the β-phosphate of the bound nucleotide and is thought to facilitate catalysis through this coordination.
Note that substitutions at this lysine disrupt nucleotide binding. Even substitution to arginine creates a pseudokinase, as in the
well-characterized KSR family. Mutation of the Lys to an alaphatic (e.g. to Met) is a common
kinase-dead allele. Note that such mutations block nucleotide binding, which can radically alter protein stability in the cell, and
may therefore result in phenotypes that are independent of kinase activity. Remarkably, the well-conserved
WNK (With-No-Lys) family of kinases have migrated their VAIK-Lys to β-sheet 2 and
retain kinase activity in spite of a substitution that
would otherwise render them pseudokinases. The WNK kinases are
regulated by Na+ binding in the active site,
The alanine in the motif is also important, as it points towards the purine ring. Substitution to larger residues disrupts
nucleotide binding, though valine is often tolerated. Note that the
WNG family require larger residues (e.g. Leu) at this position,
likely due to their lack of Gly-loop.
The VAIK-Lys is coordinated by a conserved glutamate in the α-C helix (green), which forms a
strong salt bridge upon nucleotide-binding. This interaction requires rotation of the α-C helix in many kinases, and the
resulting conformational change is thought to be a major point of regulation of kinase activation.
Note that the pocket resulting from α-C helix rotation is the site of binding of a class of
conformationally selective, non-competitive MEK1/2 inhibitors.
The HRD motif
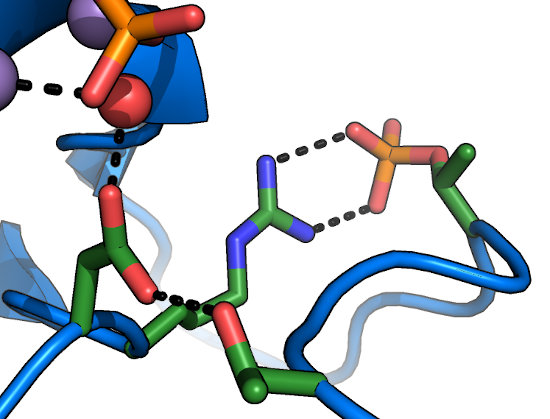
The catalytic base of the active site is formed by the carboxylate of the Asp in the "HRDLKPEN" motif. In an activated
kinase, the Asp held in place by the sidechain hydroxyl of the GT-motif Thr (which lies at the end of the activation loop). While there have been controversial
reports of kinases with substitutions at this position (e.g. to Asn) retaining activity, this residue is usually
thought to be absolutely required for phosphoryl transfer, and is an excellent site to mutate for a kinase-dead allele that does not affect
nucleotide-binding. The HRD-Arg is conserved in kinases activated by phosphorylation on their activation loops, and recognizes the phosphorylated
residue at this site to lock the activation loop in place (Ser/Thr or Tyr).
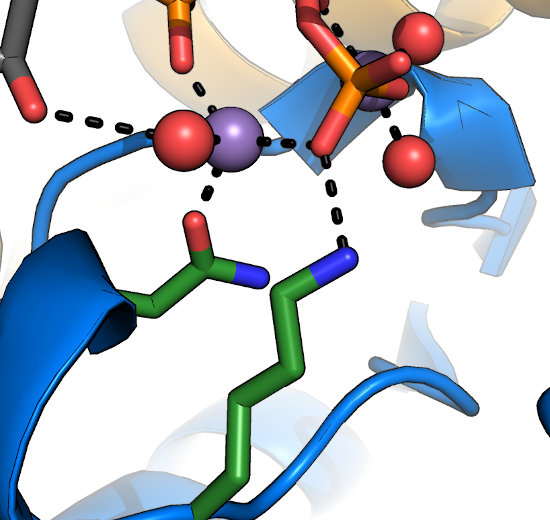
Often overlooked are the conserved Lys and Asn in the HRDLKPEN motif. The Lys recognizes the γ-phosphate
of nucleotide and stabilizes it during transfer. The Asn coordinates one of the two divalent ions (typically Mg2+) required for nucleotide-binding
and kinase activity. In general, mutation at either of these sites would be expected to disrupt kinase activity. In the metazoan
Tyrosine Kinase (TK), however, the motif has reorganized to HRDLAARN, where
the second Arg serves the role of the Lys in other kinases. Note that while this altered HRD motif is a hallmark of the TK family, these substitutions are not
required for phosphorylation on Tyr residues.
The DFG motif
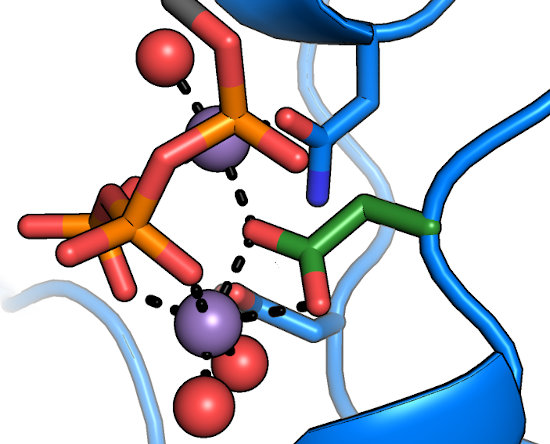
The aspartate of the DFG motif (green) is understood to be central to nucleotide binding and catalysis, as it coordinates
both of the divalents in the active site. Any substitutions at this position result in a catalytically inactive protein.
Intriguingly, the "DFG+1" residue has been implicated
as a major determinant in Ser/Thr specificity; large bulky residues at this position
(e.g. Phe) lead to preference for Ser, while smaller residues
(Leu, Ser, etc.) lead to a preference for Thr as the phosphoacceptor.
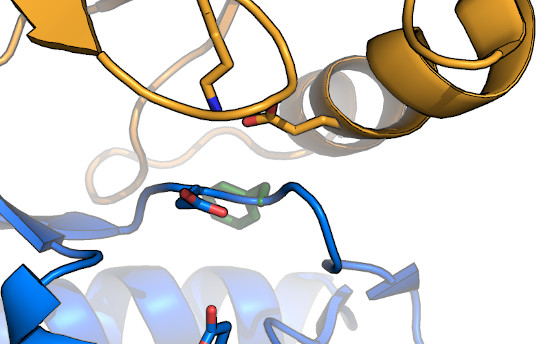
The DFG-flip: The structure of imatinib bound to
Abl1FPU revealed that the drug bound in a conformation in which the
Phe of the DFG-motif had "flipped" from the typical inward-facing conformation, to an outward-facing conformation that was incompatible with
nucleotide-binding. Inhibitors that target this conformation are referred to as "Type II" inhibitors (as opposed to DFG-in inhibitors, which are
"Type I"). While it was originally thought that kinases such as Abl were more energetically able to adopt the DFG-out conformation, further
work clarified that the DFG-flip is coupled to regulation of kinase activation,
both in Abl, and in other kinases, as well. In general, the Phe and Gly
of the DFG are relatively well conserved, likely to enable the DFG-flip. In addition, substitution of the Gly to any other residue results in the
side-chain oriented towards bound nucleotide, which would be expected to alter nucleotide affinity. Notably,
some pseudokinases, such as RNAse L and
the active kinases in the
apicomplexan WNG family appear to use Asp or Glu at this position to coordinate Mg2+.
Other miscellaneous motifs
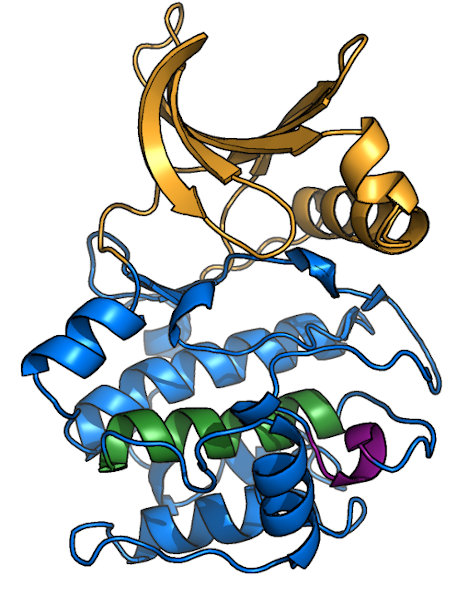
There are several other well-conserved motifs in the kinase fold, though most are associated with stabilizing the overall structure rather than
playing a catalytic or regulatory role. Examples include the DFW-helix (green), which forms the core
of the C-lobe, and the APE-motif (purple)
that anchors the C-terminal end of the activation loop to the C-lobe structure. Also notable it the GT-motif lies just N-terminal to the
APE-motif and serves to stabilize the HRD-Asp for catalysis (see "HRD-motif" tab).
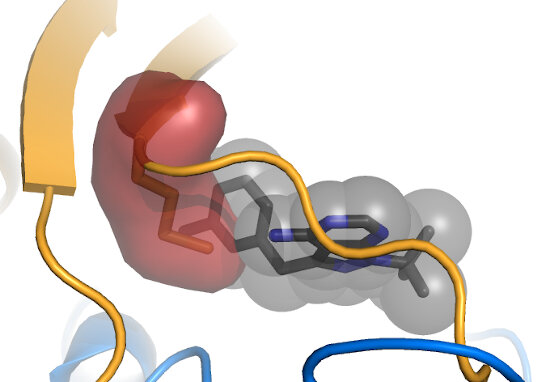
The "Gatekeeper" position is usually occupied by a bulky amino acid (red), and can be mutated to
a smaller residue, such as Gly or Ala (green) to create a kinase that is specifically susceptible to inhibition by an ATP analog with a bulky moiety (black).
Shokat and colleagues pioneered this method for both allele-specific inhibition,
as well as for allele-specific ATP analogs, including ATPγS. Note that
some kinases, such as members of the CDPK family, natively have small gatekeepers, and can those be
inhibited by "bumped" analogs. Also note that mutation at the gatekeeper position can alter kinase activity. Often mutation results in some
reduction in activity, though
ERK1/2 become constitutively activated by a small gatekeeper mutation.